In this blog, we have Dr. Yuanwei Liu, the winner of 2021 IEEE ComSoc Outstanding YP Award Nominee, sharing an insightful story about his research experiences.
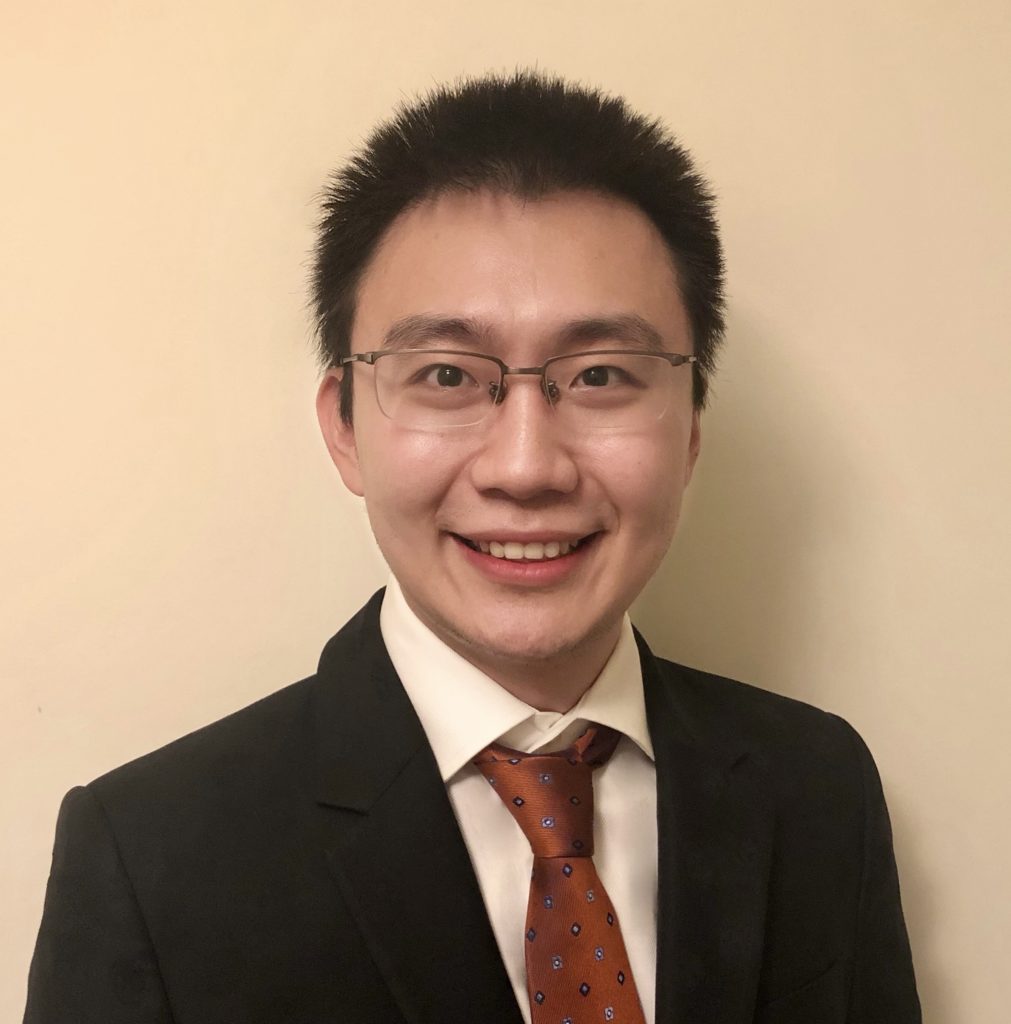
Yuanwei Liu is an Associate Professor in the School of Electronic Engineering and Computer Science at Queen Mary University of London (QMUL), London, U.K. (Aug. 2021-present), where he started as an Assistant Professor in Sep. 2017. He currently serves as a Senior Editor of IEEE Communications Letters, an Editor of IEEE Transactions on Wireless Communications and IEEE Transactions on Communications. He is an IEEE Vehicular Technology Distinguished Lecturer and a Senior Member of the IEEE. He is the founding chair of the IEEE ComSoc Next Generation Multiple Access Emerging Technologies Initiative. Dr. Liu is the recipient of the 2021 Web of Science Highly Cited Researcher, 2020 IEEE ComSoc Outstanding Young Researcher Award for the EMEA Region, 2020 Early Achievement Award of the IEEE ComSoc SPCC Technical Committee, and 2021 IEEE ComSoc CTTC Early Achievement Awards. For more details, please check Yuanwei Liu’s homepage.
Introduction
Recently, intelligent surfaces are regarded as one of the most promising techniques in wireless communications for beneficially adjusting the propagation of radio waves. This blog discusses one revolutionary category of intelligent surfaces, namely, simultaneously transmitting and reflecting (STAR) reconfigurable intelligent surfaces (RISs). But before that, I’d like to start with how our team came up with the idea.
STAR-RISs: My story of incubating a new idea
Recently, the idea of controlling the wireless signal during propagation began flourishing. Exploiting the recent advancement in metasurfaces and the corresponding fabrication technologies, the concept of intelligent surfaces was proposed, e.g., RISs and intelligent reflecting surfaces (IRSs). Intelligent surfaces are two-dimensional structures comprising a large number of low-cost reconfigurable elements. However, the pioneering works on intelligent surfaces mostly focused on the single function for manipulating signals, namely reflection or transmission, which results in a half-space coverage. In practice, there are many application scenarios which demand full-space coverage. For example, the indoor-to-outdoor connectivity for IoT networks and extended reality (XR) networks. As a result, my team started to incubate ideas that could realize a 360° smart radio environment.
One day, an idea occurred to me when I was staring through my window, contemplating this problem. What about we introduce both controllable reflection and transmission (refraction) simultaneously? Just like the glass window, which allows us to see our reflection and the things outside, a STAR-RIS should be able to transmit and reflect the incident wireless signal to both sides and thus, enables full-space coverage. The key issue left to be solved is how to achieve the dynamic control on both the transmission and reflection coefficients at each STAR-RIS element.
To fill this knowledge gap, my team and I turned to physics, specifically electromagnetic theory. Fortunately, we could confirm that simultaneous wave manipulation of both transmitted and reflected waves is achievable through configuring the surface’s equivalent electric and magnetic impedances.
Components | Reflecting-Only RIS | STAR-RIS |
Substrates | Opaque | Transparent at radio frequency |
Elements | Only support electric currents | Support both electric and magnetic currents |
Coefficients | Reflection coefficients | Transmission and reflection coefficients |
To make an analogy, for reflecting-only RISs, the reconfigurable elements on the substrate are like biscuits placed on a metal plate, while for STAR-RISs, the reconfigurable elements are like ice cubes in a glass of water. In terms of implementations, there are several potential options and existing prototypes. For the periodic structure of the STAR-RIS, we can loosely classify their hardware implementations into two categories: patch-array-based and metasurface-based implementations. As illustrated in the following figure, the patch-array-based implementations consist of periodic cells with sizes of a few centimetres. Because of their relatively large sizes, each cell (patch) can be made tunable by incorporating either PIN diodes or delay lines. By contrast, the metasurface-based implementations have periodic cells on the order of a few millimetres, possibly micrometres, or even molecular sizes. Hence, they require more sophisticated controls of their EM properties, such as conductivity and permittivity.
STAR-RISs in 6G networks
Our next step was to employ STAR-RISs in wireless communication systems to determine their performance limits and optimization algorithms. First of all, we established the hardware and signal models for STAR-RIS. This modelling process took longer than we anticipated. Initially, we modelled the phase-shift for the reflection and transmission coefficients to be independent of each other, as illustrated in the following figure (Fig. 2). However, we found such an independent control difficult and costly to achieve in practice.
As a further advance, we proposed several more low-cost and easy-to-implement models for STAR-RISs in our following research publications. For example, we investigate the correlation between the transmission and reflection coefficients and propose a coupled phase-shift model for STAR-RISs. We also proposed models for the dual-sided STAR-RIS, where the wireless signals can be simultaneously incident on both sides of the STAR-RIS. Based on these models, we can investigate the performance of STAR-RIS. Just to give the readers a quick impression of the improved coverage of STAR-RIS, we include the following simulation figure (Fig. 3). As can be observed in the figure, the power density of the STAR-RIS (b) is significantly higher near the target angles, forming a beam-like radiation pattern. In contrast, the transmitted and reflected beams of the conventional RIS (c) are less focused with weaker channel gains. For details, please check out our IEEE Communications Letters popular paper co-authored with my PhD student Jiaqi Xu.
Moreover, for smoothly employing STAR-RISs in wireless communications, our team further proposed three practical operating protocols, namely energy splitting (ES), mode switching (MS), and time switching (TS), and developed the corresponding efficient joint beamforming method. For details, please check out our popular IEEE Transactions on Wireless Communications paper .
Last but not least, the promising applications of STAR-RISs in 6G networks are diverse. The figure below illustrates the potential application scenarios of STAR-RISs in wireless communications for both outdoor, indoor, and outdoor-to-indoor environments. In outdoor communications, similar to conventional reflecting-only RISs, STAR-RISs can be mounted on building facades and roadside billboards to create an additional communication link. More innovatively, STAR-RISs can also be accommodated by the windows of vehicles. For outdoor-to-indoor communications, the severe penetration loss caused by building walls gravely restricts the coverage provided by outdoor BSs, especially in mmWave and THz communications. For indoor communications, STAR-RISs are more appealing than conventional reflecting-only RISs. As conventional reflecting-only RISs merely achieve half-space coverage, the signals emerging from the AP may require multi-hop bounces to reach the target user. However, by exploiting both transmission and reflection, the resultant full-space coverage may reduce the propagation distance, thus increasing the received signal power [paper link].
Conclusions
In post-epidemic times, the importance of network communication technology has become more prominent, and increasingly complicated network interaction scenarios have put forward requirements for the development of 6G technology. Taking research activities as an example, more and more reports, tutorials, and even academic conferences are held in the form of Zoom. By moving from offline physical space to online space, we can improve the efficiency of communication and broadens the scope of communication. In this setting, new physical layer technology like STAR-RISs serves as the key enabler for providing low-cost, energy-efficient and ubiquitous coverage communications.
Related papers:
[R1] Y. Liu et al., “STAR: Simultaneous Transmission and Reflection for 360° Coverage by Intelligent Surfaces,” in IEEE Wireless Communications, vol. 28, no. 6, pp. 102-109, December 2021, doi: 10.1109/MWC.001.2100191.
[R2] J. Xu, Y. Liu et al., “Simultaneously Transmitting and Reflecting Intelligent Omni-Surfaces: Modeling and Implementation,” in IEEE Vehicular Technology Magazine, vol. 17, no. 2, pp. 46-54, June 2022, doi: 10.1109/MVT.2022.3157069.
[R3] J. Xu, Y. Liu, X. Mu and O. A. Dobre, “STAR-RISs: Simultaneous Transmitting and Reflecting Reconfigurable Intelligent Surfaces,” in IEEE Communications Letters, vol. 25, no. 9, pp. 3134-3138, Sept. 2021, doi: 10.1109/LCOMM.2021.3082214.
[R4] X. Mu, Y. Liu, L. Guo, J. Lin and R. Schober, “Simultaneously Transmitting and Reflecting (STAR) RIS Aided Wireless Communications,” in IEEE Transactions on Wireless Communications, vol. 21, no. 5, pp. 3083-3098, May 2022, doi: 10.1109/TWC.2021.3118225.
**Statements and opinions given in this blog are the expressions of the contributor(s). Responsibility for the content of published articles rests upon the contributor(s), not on the IEEE Communication Society or the IEEE Communications Society Young Professionals.